One of the fundamental ingredients for future gravitational wave observatories, such as the Einstein Telescope, will be the development of sophisticated ultra-high vacuum technologies. The need to detect the extremely weak spacetime perturbations induced by the passage of a gravitational wave inside interferometers requires the realization of a vacuum system that eliminates as much as possible not only air, but also other possible sources of disturbance (which can mimic a gravitational signal), such as vibrations, dust, or electromagnetic effects.
To best tackle this difficult challenge, in 2022 the Einstein Telescope scientific collaboration entered into a collaboration agreement with CERN, which has been at the forefront for decades in developing ultra-high vacuum systems for particle accelerators, particularly the Large Hadron Collider (LHC). It is an unprecedented collaboration and a growth opportunity for both parties involved: on one hand, ET can rely on the experience of some of the world’s leading experts in vacuum technologies, and on the other hand, CERN has the opportunity to engage with an ultra-high vacuum application field very different from what it typically deals with.
We discuss this with Paolo Chiggiato, head of the vacuum, surfaces, and coatings group at CERN and coordinator (together with Aniello Grado from INAF and Nick van Remortel from the University of Antwerp) of the ET vacuum system project.
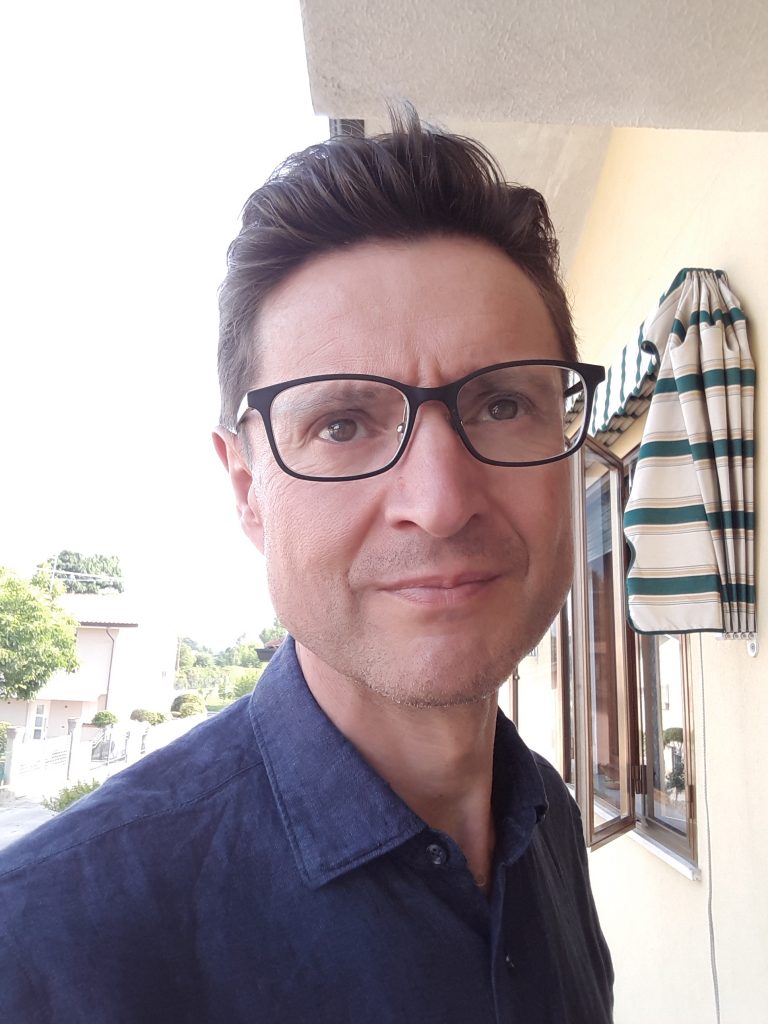
Paolo Chiggiato
CERN has been leader in developing ultra-high vacuum technologies for particle accelerators for years. Why is it so important, and what results have been achieved so far?
When you think about the concept of “creating a vacuum,” the first level of consideration is to eliminate atmospheric air as much as possible. However, this aspect does not represent the main problem in an accelerator and is relatively straightforward to address. The real challenge of ultra-high vacuum for modern particle accelerators, characterized by high-intensity beams and extremely high energies, lies in the continuous interactions between the particle beam and the surfaces it is exposed to: so, first of all, it is necessary to mitigate this dynamic effect, as well as to limit a whole series of other electromagnetic interaction sources.
For this reason, for decades, we can say that vacuum physics for accelerators has become synonymous with materials science and surface science. At CERN, the most significant progress has been made in modifying the surface of the long tubes of the LHC to minimize interactions with the particle beam: in particular, there are now six kilometers of vacuum chambers coated with special thin films based on non-evaporable getters, which ensure continuous pumping inside the accelerator, while another five kilometers will be coated with thin films of amorphous carbon, a few tens of nanometers thick, to reduce the emission of secondary electrons and the thermal load in the cryogenic system.
So far, CERN has not had a direct role in the development of vacuum technologies for gravitational wave experiments. How did the collaboration with the Einstein Telescope project come about?
As often happens, almost by chance. In January 2019, I attended a conference on vacuum and cryogenics for future gravitational wave detectors and found many interesting insights on this topic. Initially, stimulated mainly by Nobel laureate Rainer Weiss (one of the founders of the LIGO experiment, ed), I began to discuss quite regularly with the American community, engaged in designing the future gravitational wave observatory, Cosmic Explorer. But shortly after, interest and discussions also extended to the European community, and thus to the Einstein Telescope.
In the latter case, one element, in particular, favored the birth of the collaboration: the fact that ET does not yet have a defined site and involves researchers from several and different European scientific communities ensures a balance that perfectly matches the philosophy of CERN, which is an inherently impartial organization. From a more formal point of view, a fundamental step was the inclusion of ET among the experiments officially recognized by CERN, a factor that has facilitated mutual exchanges.
What are the differences in the use and impact of vacuum technologies between particle accelerators and gravitational wave interferometers?
In purely quantitative terms, the level of vacuum required is quite similar in both cases. The most significant difference lies in the fact that in an accelerator the cost of the vacuum system is never predominant, indeed in most cases it is a small fraction of the total cost, dominated by civil engineering, magnets, or acceleration systems. On the contrary, in an experiment for gravitational wave research, vacuum is one of the highest cost items. This is especially true for future experiments, such as the Einstein Telescope, because the cost of the vacuum system typically scales with the length of the interferometer arms. To get an idea of the difference, with the current Virgo and LIGO experiments, we deal with a total length ranging from 6 to 16 kilometers, while with the Einstein Telescope, it will increase to 120 kilometers, and for the Cosmic Explorer even to 160 kilometers. In the case of ET, vacuum will be the second-highest cost item, amounting to over half a billion euros, second only to the expenditure necessary for the construction of underground infrastructures.
What implications does this last point have?
From my point of view, it is a very interesting and fascinating challenge, different from what we are used to handling in the context of the LHC. In this case, the need to optimize costs makes it mandatory to evaluate and plan production processes as a whole, dividing them into different work packages: not only the more “classic” aspects such as vacuum technology development, mechanical engineering, and surface treatments but also transportation, logistics, and installation become fundamental components of management to reduce costs and increase efficiency.
More specifically, what is CERN’s commitment, and how long will it last?
The main goals are twofold: to write the technical design report (TDR) of the Einstein Telescope’s vacuum system, scheduled for delivery by the end of 2025, and to build a pilot sector at CERN, approximately 45 meters long, to directly test some technological solutions for the vacuum system that we will propose. At the moment, the pilot sector is in the design phase: the goal is to have all the mechanical components available by the end of this year and to start installation, while next year will be dedicated to data collection and to the TDR writing. Once the experiment site is decided, our task will end after a transition phase, during which we will hand over to the ET working group, which will be responsible for implementing the system directly at the chosen site.
Credits featured image: CERN